Driving Industrial E-Mobility With Silicon Carbide Semiconductors
Article
Introduction
Governments and other organizations around the world are attempting to curb greenhouse gas emissions, including carbon dioxide (CO2), by implementing increasingly stricter regulations and by offering incentives for energy suppliers, manufacturers, and consumers to adopt alternative energy technologies. In the case of energy generation, for example, power companies are increasingly turning to renewable energy, which refers to energy from a natural, self-replenishing source that won’t run out. Examples are solar energy, hydroelectric (including tidal energy), and wind power.
One of the largest sources of greenhouse gases is the transportation market, which accounts for approximately 20% of global CO2 emissions. The easiest and fastest way to reduce these emissions is to switch from powering vehicles using fossil fuels like diesel, gasoline, and oil to powering them using electricity in the form of batteries, hydrogen fuel cells, or related technologies.
Industrial E-Mobility
The deployment of consumer and fleet electric vehicles (EVs) is now well underway. According to the International Energy Agency (IEA),2 electric cars accounted for around 18% of all cars sold in 2023, up from only 2% five years earlier in 2018.
Following closely behind the adoption of consumer and fleet EVs, new transportation markets are transitioning to electric mobility (or e-mobility) to achieve a sustainable, CO2-neutral future.
From commercial, construction, and agricultural vehicles to aircraft, marine vessels, and trains, new electrified vehicle concepts are emerging around the world.
The term “industrial e-mobility” refers to the entire range of industrial applications and vehicles across land (roads and railways), sky, and water that already run on—or are in the process of adopting—electric platforms.3
Three of the biggest challenges for the future of industrial e-mobility are as follows:
- The availability of appropriate energy sources to power the vehicles, such as batteries, hydrogen (as fuel), and hydrogen fuel cells.
- The availability of systems and devices to control this energy, such as transforming direct current (DC) from a battery into alternating current (AC) for use by a motor.
- The availability of any necessary supporting infrastructure, including electric grid capacity, high power charging stations, and hydrogen refueling stations.
Industrial E-Mobility Power Electronics
One of the main systems in an industrial e-mobility vehicle is the power inverter (also known simply as the inverter), which transforms power provided by a DC source such as the battery in an EV into the AC power used to drive the motors. In addition to handling high currents and voltages, these devices and systems are often required to operate in hostile environments.
Along with main propulsion inverters, industrial e-mobility applications integrate power electronics within many other systems, including battery management systems (BMS), auxiliary power supplies, auxiliary motor drives, pump and fan actuators (HVAC systems), and onboard chargers. Each electrified system further reduces the number of mechanical components compared to their internal combustion engine (ICE) counterparts, enabling greater efficiency, lighter weight, and lower total cost of ownership (TOC).
These electrified systems conserve system-wide energy usage, reduce emissions, and extend transportation vehicle lifetimes through low losses, high power density, high reliability, and robustness.
Why Silicon Carbide?
Conventional semiconductors like silicon (Si) and gallium arsenide (GaAs) have a bandgap in the range of 0.6 to 1.5 electronvolt (eV). By comparison, wide-bandgap (WGB) semiconductors like silicon carbide (SiC) have bandgaps of 2 eV or higher. WGB semiconductors can operate at much higher voltages, higher switching frequencies, and higher temperatures than their conventional semiconductor cousins. For example, silicon carbide has approximately 10X higher dielectric breakdown field strength in comparison to silicon technology.
Silicon carbide is widely recognized as being the best-in-class technology for the voltage classes required by industrial e-mobility applications, not only in the main power inverter, but also in the wide range of auxiliary power supplies and drives found in these applications.
Overall, silicon carbide enables higher power, greater efficiency, lower switching losses, and higher switching frequency, all within a lighter weight, smaller system size that results in a lower total cost of ownership (TCO).
Why Wolfspeed?
Wolfspeed is an American developer and manufacturer of wide-bandgap semiconductors, focused on silicon carbide materials and devices for e-mobility, renewable energy, and industrial power applications.
In the case of industrial e-mobility, applications must manage high voltages and high currents—often under demanding environmental conditions—efficiently and reliably. As was previously noted, compared to silicon, silicon carbide devices enable higher switching frequencies and greater power densities at much higher operating temperatures – which are all necessary for high-power industrial e-mobility. Industrial e-mobility includes, but is not limited to the following:
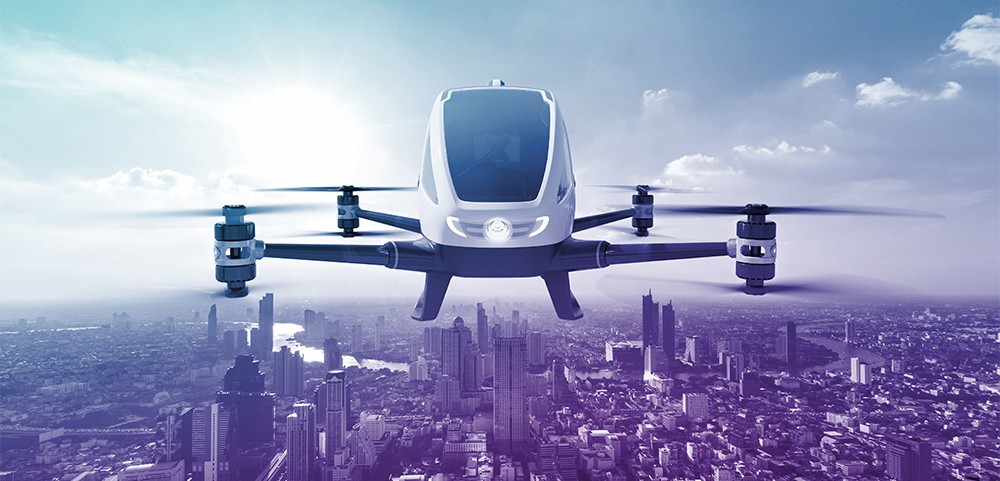
Sky
Electrified sky applications include electric vertical takeoff and landing (eVTOL), electric short take-off and landing (eSTOL), electric conventional take-off and landing (ECTOL), and a high efficiency architecture known as more electric aircraft (MEA)
Any industrial e-mobility power system that must handle high voltages and currents in demanding conditions can benefit from Wolfspeed’s state-of-the-art silicon carbide offerings. These systems include main inverters, fuel cell boost converters, battery management systems, auxiliary power supply units, auxiliary power drives, pump and fan actuators, on-board chargers, renewable energy conversion, and high-power conversion.
Through its unrivaled expertise and capacity, Wolfspeed is leading the transformation from silicon to silicon carbide, shaping the future of a wide variety of semiconductor markets, including the transition to electric vehicles in general, and industrial e-mobility in particular, the evolution of renewable energy and energy storage, and the advancement of industrial applications.
Conclusion
The world is facing a climate crisis, which is primarily caused by human generation of greenhouse gasses like carbon dioxide. Around one fifth of carbon dioxide is generated by the transportation market. Governments and other organizations are attempting to curb these emissions by reducing the use of fossil fuels and increasing the deployment of electric technologies.
Electronic control systems implemented using wide-bandgap semiconductor materials like silicon carbide can operate at much higher voltages, higher switching frequencies, and higher temperatures than conventional semiconductor materials like silicon.
Wolfspeed introduced the world’s first commercially available, fully qualified silicon carbide MOSFET. For more than 35 years, Wolfspeed has been vertically integrated and making silicon carbide for use in a range of power applications such as those presented by industrial e-mobility markets.